-
Global Warming and Changing the Range of Seasonal Temperatures
- Baseline Seasonal Temperature Range
- Seasonal Temperature Shifts Since 1900
- Global Warming Since 1900: Winter (Northern Hemisphere)
- Global Warming Since 1900: Spring (Northern Hemisphere)
- Global Warming Since 1900: Summer (Northern Hemisphere)
- Global Warming Since 1900: Fall (Northern Hemisphere) Global Warming and Permafrost Melt
- Permafrost Melt Since 1900
- Area of Permafrost Melt Since 1900
Global Warming and Changing the Range of Seasonal Temperatures
Baseline Seasonal Temperature Range
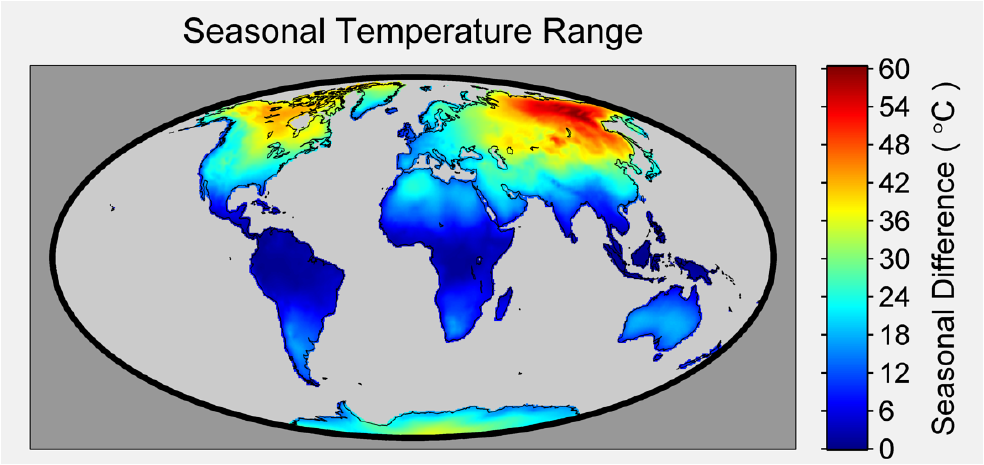
Seasonal Temperature Shifts Since 1900

Global Warming Since 1900: Winter (Northern Hemisphere)

Global Warming Since 1900: Spring (Northern Hemisphere)

Global Warming Since 1900: Summer (Northern Hemisphere)

Global Warming Since 1900: Fall (Northern Hemisphere)

Global Warming and Permafrost Melt
Permafrost Melt Since 1900

Area of Permafrost Melt Since 1900
